Cardiac Arrhythmias: Difference between revisions
No edit summary |
|||
(10 intermediate revisions by one other user not shown) | |||
Line 1: | Line 1: | ||
''Sébastien Krul, MD'' | ''Sébastien Krul, MD'' | ||
=Introduction= | |||
= | |||
A basic knowledge of the cardiac action potential and cardiac conduction system facilitates understanding of cardiac arrhythmias. The effects and side-effects of anti-arrhythmic drugs are depended on the influence on ion channels involved in the generation and/or perpetuation of the cardiac action potential. | A basic knowledge of the cardiac action potential and cardiac conduction system facilitates understanding of cardiac arrhythmias. The effects and side-effects of anti-arrhythmic drugs are depended on the influence on ion channels involved in the generation and/or perpetuation of the cardiac action potential. | ||
==Cardiac Action Potential== | ==Cardiac Action Potential== | ||
The cardiac action potential is a result of ions flowing through different ion channels. Ion channels are passages for ions (mainly Na<sup>+</sup>, K<sup>+</sup>, Ca<sup>2+</sup> and Cl<sup>-</sup>) that facilitate movement through the cell membrane. Changes in the structure of these channels can open, inactivate or close these channels and thereby control the flow of ions into and out of the myocytes. Due to differences in the type and structure of ion channels, the various parts of the heart have slightly different action potential characteristics. Ion channels are mostly a passive passageway, where movement of ions is caused by the electrochemical gradient. In addition to these passive ion channels a few active trigger-dependent channels exist that open or close in response to certain stimuli (for instance acetylcholine or ATP). The changes in the membrane potential due to the movement of ions produce an action potential which lasts only a few hundreds of milliseconds. Disorders in single channels can lead to arrhythmias, as seen in the section [[Primary_Arrhythmias]]. The action potential is propagated throughout the myocardium by the depolarization of the immediate environment of the cells and through intracellular coupling with gap-junctions.<cite>Kleber</cite> | The cardiac action potential is a result of ions flowing through different ion channels. Ion channels are passages for ions (mainly Na<sup>+</sup>, K<sup>+</sup>, Ca<sup>2+</sup> and Cl<sup>-</sup>) that facilitate movement through the cell membrane. Changes in the structure of these channels can open, inactivate or close these channels and thereby control the flow of ions into and out of the myocytes. Due to differences in the type and structure of ion channels, the various parts of the heart have slightly different action potential characteristics. Ion channels are mostly a passive passageway, where movement of ions is caused by the electrochemical gradient. In addition to these passive ion channels a few active trigger-dependent channels exist that open or close in response to certain stimuli (for instance acetylcholine or ATP). The changes in the membrane potential due to the movement of ions produce an action potential which lasts only a few hundreds of milliseconds. Disorders in single channels can lead to arrhythmias, as seen in the section [[Primary_Arrhythmias|primary arrhythmias]]. The action potential is propagated throughout the myocardium by the depolarization of the immediate environment of the cells and through intracellular coupling with gap-junctions.<cite>Kleber</cite> | ||
In summary during the depolarization, sodium ions (Na<sup>+</sup>) stream into the cytoplasm of the cell followed by a influx of calcium (Ca<sup>2+</sup>) ions (both from the inside (sarcoplasmatic reticulum) and outside of the cell). These Ca<sup>2+</sup> ions cause the actual muscular contraction by coupling with the muscle fibers. During repolarization the cell returns to the resting membrane potential, due to the passive efflux of K<sup>+</sup>(Figure 1). In detail the (ventricular) action potential can be divided in five phases: <Cite>Berne,Braunwald</Cite> | In summary during the depolarization, sodium ions (Na<sup>+</sup>) stream into the cytoplasm of the cell followed by a influx of calcium (Ca<sup>2+</sup>) ions (both from the inside (sarcoplasmatic reticulum) and outside of the cell). These Ca<sup>2+</sup> ions cause the actual muscular contraction by coupling with the muscle fibers. During repolarization the cell returns to the resting membrane potential, due to the passive efflux of K<sup>+</sup>(Figure 1). In detail the (ventricular) action potential can be divided in five phases: <Cite>Berne,Braunwald</Cite> | ||
Line 49: | Line 46: | ||
===Ventricle=== | ===Ventricle=== | ||
The ventricle is activated through the dense network of Purkinje fibers originating from the bundle branches. They penetrate the myocardium and are the starting point of the ventricular activation. The left ventricular areas first excited are the anterior and posterior paraseptal wall and the central left surface of the interventricular septum. The last part of the left ventricle to be activated is the posterobasal area. Septal activation starts in the middle third of the left side of the interventricular septum, and at the lower third at the junction of the septum and posterior wall. Activation of the right ventricle starts near the anterior papillary muscle 5 to 10 | The ventricle is activated through the dense network of Purkinje fibers originating from the bundle branches. They penetrate the myocardium and are the starting point of the ventricular activation. The left ventricular areas first excited are the anterior and posterior paraseptal wall and the central left surface of the interventricular septum. The last part of the left ventricle to be activated is the posterobasal area. Septal activation starts in the middle third of the left side of the interventricular septum, and at the lower third at the junction of the septum and posterior wall. Activation of the right ventricle starts near the anterior papillary muscle 5 to 10 milliseconds after onset of the left ventricle.<cite>Durrer</cite> | ||
=Mechanisms of Arrhythmia= | =Mechanisms of Arrhythmia= | ||
Line 55: | Line 52: | ||
[[File:Mechanisms.svg|right|thumb|400px|'''Figure 3.''' The different mechanisms of arrhythmia.]] | [[File:Mechanisms.svg|right|thumb|400px|'''Figure 3.''' The different mechanisms of arrhythmia.]] | ||
Structural abnormalities or electric changes in the cardiomyocytes can impede impulse formation or change cardiac propagation, therefore facilitating arrhythmias. Arrhythmogenic mechanisms can arise in single cells (automaticity, triggered activity), but other mechanisms require multiple cells for arrhythmica induction (re-entry). We briefly discuss the | Structural abnormalities or electric changes in the cardiomyocytes can impede impulse formation or change cardiac propagation, therefore facilitating arrhythmias. Arrhythmogenic mechanisms can arise in single cells (automaticity, triggered activity), but other mechanisms require multiple cells for arrhythmica induction (re-entry). We briefly discuss the pathophysiological mechanisms of the main causes of arrhythmia. <Cite>Coronel</Cite> | ||
== Abnormal Impulse Formation== | == Abnormal Impulse Formation== | ||
Line 63: | Line 60: | ||
===Triggered Activity=== | ===Triggered Activity=== | ||
Triggered activity is | Triggered activity is depolarization of a cell triggered by a preceding activation. Due to early or delayed afterdepolarizations the membrane potential depolarizes and, when reaching a threshold potential, activates the cell. These afterdepolarizations are depolarizations of the membrane potential initiated by the preceding action potential. Depending on the phase of the action potential in which they arise, they are defined as early or late afterdepolarizations (figure 3). | ||
* A disturbance of the balance in influx and efflux of ions during the plateau phase (phase 2 or 3) of the action potential is responsible for the early afterdepolarizations. Multiple ion currents can be involved in the formation of early after depolarizations depending on the triggering mechanism. Early afterdepolarizations can develop in cells with an increased duration of the repolarization phase of the action potential, as the plateau phase is prolonged. | * A disturbance of the balance in influx and efflux of ions during the plateau phase (phase 2 or 3) of the action potential is responsible for the early afterdepolarizations. Multiple ion currents can be involved in the formation of early after depolarizations depending on the triggering mechanism. Early afterdepolarizations can develop in cells with an increased duration of the repolarization phase of the action potential, as the plateau phase is prolonged. The prolonged repolarization might reactivate the Ca2+ channels that have recovered from activation at the beginning of the repolarization. Otherwise disparity in action potential duration of surrounding myocytes can destabilize the plateau phase through adjacent depolarizing currents. | ||
* Delayed afterdepolarizations occur after the cell has recovered after completion of repolarization. In delayed afterdepolarization an abnormal Ca<sup>2+</sup> handling of the cell is responsible for the afterdepolarizations due to release of Ca<sup>2+</sup> from the storage of Ca<sup>2+</sup> in the sarcoplasmatic reticulum. The accumulation of Ca<sup>2+</sup> increases membrane potential and depolarizes the cell until it reaches a certain threshold, thereby creating an action potential. A high heart rate can result in the accumulation of intracellular Ca<sup>2+</sup> and induce delayed afterdepolarizations. | * Delayed afterdepolarizations occur after the cell has recovered after completion of repolarization. In delayed afterdepolarization an abnormal Ca<sup>2+</sup> handling of the cell is responsible for the afterdepolarizations due to release of Ca<sup>2+</sup> from the storage of Ca<sup>2+</sup> in the sarcoplasmatic reticulum. The accumulation of Ca<sup>2+</sup> increases membrane potential and depolarizes the cell until it reaches a certain threshold, thereby creating an action potential. A high heart rate can result in the accumulation of intracellular Ca<sup>2+</sup> and induce delayed afterdepolarizations. | ||
Line 72: | Line 69: | ||
===Re-entry=== | ===Re-entry=== | ||
Re-entry or circus movement is a | Re-entry or circus movement is a multicellular mechanism of arrhythmia. Important criteria for the development of re-entry are a circular pathway with an area in this circle of unidirectional block and a trigger to induce the re-entry movement. Re-entry can arise when an impulse enters the circuit, follows the circular pathway and is conducted through an unidirectional (slow conducting) pathway. Whilst the signal is in this pathway the surrounding myocardium repolarizes. If the surrounding myocardium has recovered from the refractory state, the impulse that exits the area of unidirectional block can reactivate this recovered myocardium. This process can repeat itself and thus form the basis of a re-entry tachycardia. Slow conduction and/or a short refractory period facilitate re-entry. The reason of unidirectional block can be anatomical ([[Tachycardia|atrial flutter, AVNRT, AVRT]]) or functional (myocardial ischemia) or a combination of both.<Cite>deBakker,Janse</Cite> | ||
=References= | =References= |
Latest revision as of 13:03, 9 May 2013
Sébastien Krul, MD
Introduction
A basic knowledge of the cardiac action potential and cardiac conduction system facilitates understanding of cardiac arrhythmias. The effects and side-effects of anti-arrhythmic drugs are depended on the influence on ion channels involved in the generation and/or perpetuation of the cardiac action potential.
Cardiac Action Potential
The cardiac action potential is a result of ions flowing through different ion channels. Ion channels are passages for ions (mainly Na+, K+, Ca2+ and Cl-) that facilitate movement through the cell membrane. Changes in the structure of these channels can open, inactivate or close these channels and thereby control the flow of ions into and out of the myocytes. Due to differences in the type and structure of ion channels, the various parts of the heart have slightly different action potential characteristics. Ion channels are mostly a passive passageway, where movement of ions is caused by the electrochemical gradient. In addition to these passive ion channels a few active trigger-dependent channels exist that open or close in response to certain stimuli (for instance acetylcholine or ATP). The changes in the membrane potential due to the movement of ions produce an action potential which lasts only a few hundreds of milliseconds. Disorders in single channels can lead to arrhythmias, as seen in the section primary arrhythmias. The action potential is propagated throughout the myocardium by the depolarization of the immediate environment of the cells and through intracellular coupling with gap-junctions.Kleber
In summary during the depolarization, sodium ions (Na+) stream into the cytoplasm of the cell followed by a influx of calcium (Ca2+) ions (both from the inside (sarcoplasmatic reticulum) and outside of the cell). These Ca2+ ions cause the actual muscular contraction by coupling with the muscle fibers. During repolarization the cell returns to the resting membrane potential, due to the passive efflux of K+(Figure 1). In detail the (ventricular) action potential can be divided in five phases: Berne,Braunwald
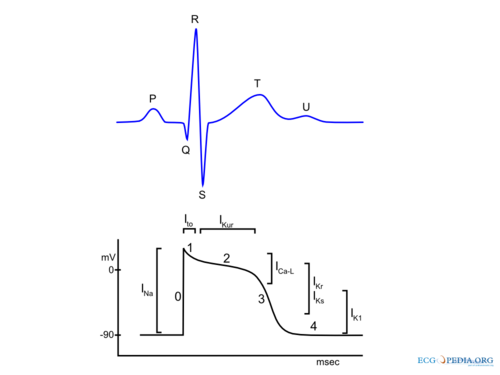
Phase 0: Rapid Depolarization
Rapid depolarization is started once the membrane potential reaches a certain threshold (about -70 to -60 mV). This produces activation of sodium channels and a rapid influx of Na+ and a corresponding rapid upstroke of the action potential. At higher potentials (-40 to -30) Ca2+ influx participates in the upstroke. In the sinus node and AV node a slower upstroke can be observed (Figure 2). This is because the rapid depolarization in these cells is mainly mediated by the slower acting Ca2+ ion channels. The slower activation produces a slower upstroke.
Phase 1: Early Rapid Repolarization
Immediately following rapid depolarization, the inactivation of the Na+ channel (INa) and subsequent activation of the outward K+ channel (Ito) and the Na+/Ca2+ exchanger (INa,Ca), which exchanges 3 Na+ for 1 Ca2+, produces a early rapid repolarization. Due to the limited role of the Na+ channel in the upstroke of sinus node and AV node cells and the subsequent slower depolarization, this rapid repolarization is not visible in their action potentials (Figure 2).
Phase 2: Plateau
The plateau phase represents an equal influx and efflux of ions in or out of the cell producing a stable membrane potential. This plateau phase is predominantly observed in the ventricular action potential. The inward movement of Ca2+ through the open L-type Ca2+ channels (ICa-L) and the exchange of Na+ for internal Ca2+ by the Na+/Ca2+ exchanger (INa,Ca) are responsible for the influx of ions during the plateau phase. The efflux of ions is the result of outward current carried by K+ (IKur and Ks).
Phase 3: Final Rapid Repolarization
Final repolarization is mainly caused by inactivation of Ca2+ channels, reducing the influx of positive ions. Furthermore repolarizing K+ currents (delayed rectifier current IKs and IKr and inwardly rectifying current IK1 and IK,Ach) are activated which increase efflux of positive K+ ions. This results in an repolarization to the resting membrane potential.
Phase 4: Resting membrane potential
During phase 4 of the action potential intracelullar and extracellular concentrations of ions are restored. Depending on cell type the resting membrane potential is between -50 to -95 mV. Sinus node and AV nodal cells have a higher resting membrane potential (-50 to -60 mV and -60 to -70 respectively) in comparison with atrial and ventricular cardiomyocytes (-80 to -90 mV). Sinus node cells and AV nodal cells (and to a lesser degree Purkinje fibers cells) have a special voltage dependent channel If, the funny current. Furthermore they lack IK1, a K+ ion channel that maintains the resting membrane potential in atrial and ventricular tissue. The If channel causes a slow depolarization in diastole, called the phase 4 diastolic depolarization, which results in normal automaticity. The frequency of the sinus node discharges are regulated by the autonomous nerve system and due to the relative high firing frequency (60-80 beats per minute) the sinus node dominates other potential pacemaker sites.
Cardiac conduction
The cardiac conduction system (Figure 2) consist of specialized fast conducting tissue though which the electric activity of the heart spreads from the atria to the ventricles. The characteristics of the different parts of the conduction system are a result of the different characteristics of the individual myocytes. On a larger level, function is controlled predominantly by the autonomic nervous system (both vagal and sympathetic nerve system). Especially the sinus node and atrioventricular node are responsive to the autonomic nerve system. The ganglionic plexus, a conglomeration of both vagal and sympathetic nerves, form the intrinsic cardiac nerve system and innervate through a network of nerve fibers the atria and ventricles. The vagal and sympathetic nerve system are both continually active in the heart, but vagal activity dominates the tonic background stimulation of the autonomic nerve system. Moreover the heart is more susceptible to vagal stimulation. Vagal stimulation provokes a rapid response and the effect dissipates swiftly in contrast to sympathetic stimulation which has a slow onset and offset. Vagal stimulation results in a reduction in sinus node activation frequency and prolongs AV nodal conduction. These effects can occur simultaneously or independent of each other. Sympathetic stimulation exerts reverse effects, accelerating the sinus node firing frequency and improving AV nodal conduction. The autonomic nerve system has a small effect on cardiomyocytes. Vagal stimulation tends to prolong the refractory period and decrease the myocardial contractility. Sympathetic stimulation has the opposite effect on the cardiac tissue. The physiological modulation of cardiac conduction is vital to adaptation of the heart to rest and exercise. However the autonomic nervous system can contribute as a modifier is certain to facilitate the occurrence of certain arrhythmias.Braunwald
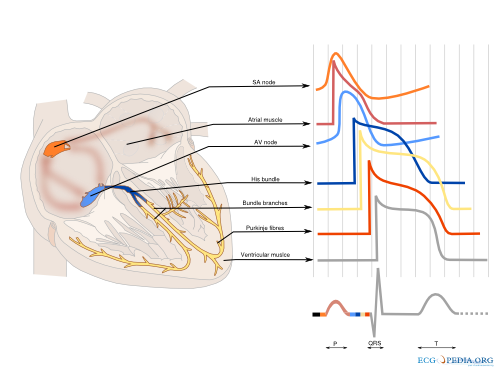
Sinus node
The sinus node is a densely innervated area located in the right atrium which is supplied by the right (55%-60%) or circumflex (40%-45%) coronary artery. It is a small structure of 10-20mm long and 2-3mm wide and contains a diversity of cells. These include pacemaker cells which are discharged synchronously due to mutual entrainment. This results in an activation wave front activating the rest of the atrium.
Atrium
The impulse formed in the sinus node is conducted through the atrium to the AV-node. Evidence indicates three preferential conduction pathways. The pathways show preferential conduction due to their anatomical structure and rather than specialized conduction properties. The three pathways are: the anterior internodal pathway, the middle internodal tract the posterior internodal pathway. The anterior internodal pathway connect to the anterior interatrial band, also known as the Bachmann bundle. This bundle of muscular tissue conducts the sinus wave front from the right to the left atrium.
AV node
The connection between atria and ventricles is facilitated through the AV node, lying in the right atrial myocardium and a penetrating part, the bundle of His. The AV node acts as a gatekeeper, regulating impulse conduction from the atrium to the ventricle. Additionally, due to the phase 4 diastolic depolarization it can exhibit impulse formation. The AV node is supplied in most cases (85%-90%) by the right coronary artery or in the remaining cases the circumflex artery.
Bundle of His
Connecting the distal AV node and the proximal bundle branches, the bundle of His is supplied by both the posterior and anterior descending coronary arteries. It is enclosed by the central fibrous body and membranous septum between the atria and the ventricles. The location and blood supply protect the bundle of His from external influences.
Bundle Branches
From the bundle of His the right bundle branch continues to the right ventricular apex. The left bundle branch splits of and divides in two fascicular branches. Commonly the left bundle branch consist of an anterior fascicle, which activates the anterosuperior portion of the left ventricle. and the thicker and more protected posterior fascicle which activates the inferoposterior part of the left ventricle.
Ventricle
The ventricle is activated through the dense network of Purkinje fibers originating from the bundle branches. They penetrate the myocardium and are the starting point of the ventricular activation. The left ventricular areas first excited are the anterior and posterior paraseptal wall and the central left surface of the interventricular septum. The last part of the left ventricle to be activated is the posterobasal area. Septal activation starts in the middle third of the left side of the interventricular septum, and at the lower third at the junction of the septum and posterior wall. Activation of the right ventricle starts near the anterior papillary muscle 5 to 10 milliseconds after onset of the left ventricle.Durrer
Mechanisms of Arrhythmia
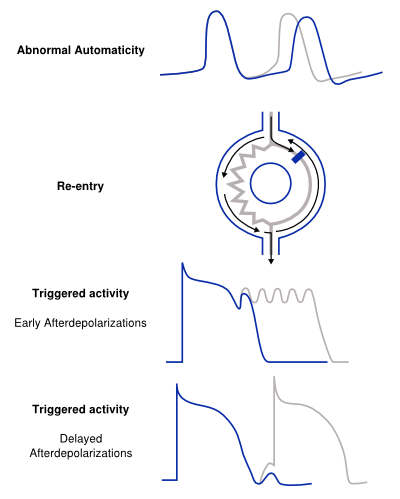
Structural abnormalities or electric changes in the cardiomyocytes can impede impulse formation or change cardiac propagation, therefore facilitating arrhythmias. Arrhythmogenic mechanisms can arise in single cells (automaticity, triggered activity), but other mechanisms require multiple cells for arrhythmica induction (re-entry). We briefly discuss the pathophysiological mechanisms of the main causes of arrhythmia. Coronel
Abnormal Impulse Formation
Abnormal Automaticity
The mechanism of abnormal automaticity is similar to the normal automaticity of sinus node cells. Abnormal automaticity can be caused by changes in the cell ion channel characteristics due to drugs (digoxine) or changes in the electrotonic environment (myocardial infarction). Abnormal automaticity can result from an increase of normal automaticity in non-sinus node cells or a truly abnormal automaticity in cells that don't exhibit a phase 4 diastolic depolarization. An important phenomenon in (both normal and abnormal) automaticity is overdrive suppression. In overdrive suppression the automaticity of cells is reduced after a period of high frequency excitation. The cellular mechanism responsible for this effect is an increased activity of the Na+, K+ pump (INa, K) which results in an increased efflux of Na+, thereby inducing a hyperpolarization.Berne
Triggered Activity
Triggered activity is depolarization of a cell triggered by a preceding activation. Due to early or delayed afterdepolarizations the membrane potential depolarizes and, when reaching a threshold potential, activates the cell. These afterdepolarizations are depolarizations of the membrane potential initiated by the preceding action potential. Depending on the phase of the action potential in which they arise, they are defined as early or late afterdepolarizations (figure 3).
- A disturbance of the balance in influx and efflux of ions during the plateau phase (phase 2 or 3) of the action potential is responsible for the early afterdepolarizations. Multiple ion currents can be involved in the formation of early after depolarizations depending on the triggering mechanism. Early afterdepolarizations can develop in cells with an increased duration of the repolarization phase of the action potential, as the plateau phase is prolonged. The prolonged repolarization might reactivate the Ca2+ channels that have recovered from activation at the beginning of the repolarization. Otherwise disparity in action potential duration of surrounding myocytes can destabilize the plateau phase through adjacent depolarizing currents.
- Delayed afterdepolarizations occur after the cell has recovered after completion of repolarization. In delayed afterdepolarization an abnormal Ca2+ handling of the cell is responsible for the afterdepolarizations due to release of Ca2+ from the storage of Ca2+ in the sarcoplasmatic reticulum. The accumulation of Ca2+ increases membrane potential and depolarizes the cell until it reaches a certain threshold, thereby creating an action potential. A high heart rate can result in the accumulation of intracellular Ca2+ and induce delayed afterdepolarizations.
Disorders of Impulse Conduction
Conduction block
Conduction block or conduction delay is a frequent cause of bradyarrhythmias, especially if the conduction block is located in the cardiac conduction system. However tachyarrhythmias can also result from conduction block when this block produces a re-entrant circuit (see below). Conduction block can develop in different (pathophysiological) conditions or can be iatrogenic (medication, surgery).
Re-entry
Re-entry or circus movement is a multicellular mechanism of arrhythmia. Important criteria for the development of re-entry are a circular pathway with an area in this circle of unidirectional block and a trigger to induce the re-entry movement. Re-entry can arise when an impulse enters the circuit, follows the circular pathway and is conducted through an unidirectional (slow conducting) pathway. Whilst the signal is in this pathway the surrounding myocardium repolarizes. If the surrounding myocardium has recovered from the refractory state, the impulse that exits the area of unidirectional block can reactivate this recovered myocardium. This process can repeat itself and thus form the basis of a re-entry tachycardia. Slow conduction and/or a short refractory period facilitate re-entry. The reason of unidirectional block can be anatomical (atrial flutter, AVNRT, AVRT) or functional (myocardial ischemia) or a combination of both.deBakker,Janse
References
<Biblio>
- Durrer pmid=5482907
- deBakker pmid=8353918
- Coronel isbn=9031348295
- Braunwald isbn=1437703984
- Kleber pmid=15044680
- Janse pmid=2678165
- Berne isbn=0815109520